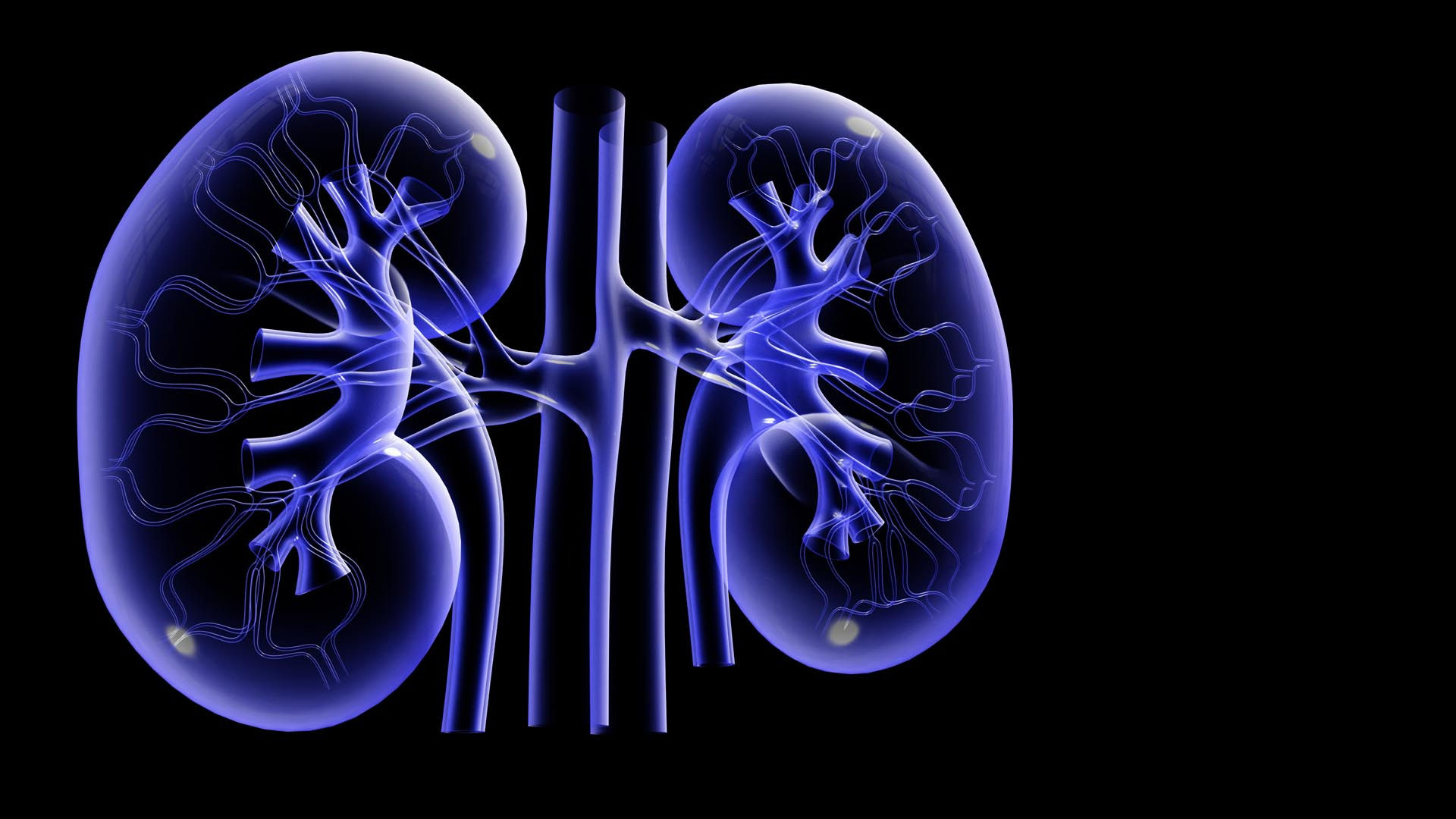
Videos and Notes for Kidney Study
This kidney physiology section provides links to many web videos that relate to each sub-topic page, along with bullet points of the main concepts provided in those videos in order to help choose which videos may be of more interest to you. The following renal physiology tutorial series are highly recommended. These two creators present the concepts from different perspectives. The Andrew Wolf videos tend to be slow, step-by-step, thorough explanations of the anatomy and physiology (these are similar to the Khan Academy videos, but with a little more detail). The Armando Hasudungan videos are faster pace, and include more details of cellular structures and functions.
Andrew Wolf – Physiology and Pathophysiology Lecture Channel
Armando Hasudungan – Biology Medicine Videos – Nephrology
The primary functions of the renal system include
-
Excretion of water-soluble waste (urea, creatinine, uric acid, bilirubin; and metabolites from hormones, paracrine signals, drugs)
-
Control of fluid volume (affects cardiac output, blood pressure), through the Renin-Angiotensin-Aldosterone System (RAAS)
-
Regulation of electrolyte balance: Na+, K+, Cl-, Pi (selective excretion/reabsorption)
-
Regulation of pH (selective excretion/reabsorption of H+ vs. HCO3-)
-
Hormone synthesis and secretion (Renin, Erythropoietin)
-
Gluconeogensis (only from protein substrates, only during starvation)
Video: Andrew Wolf, Physiology of the Renal System
www.youtube.com/watch?v=ELaXbaLKL34
The kidney is a remarkable structure of one million tubes that filter approximately 90 liters of blood plasma every day (180 liters per day total with two kidneys)*. The kidney allows the human body to recycle water and critical plasma constituents, to minimize the need for fluid intake and limit the fluid output of urine to 1.5 liters per day on average. Kidney anatomy form is driven nephron function, where the nephron is the fundamental unit of renal processes. The high rate of blood flow through the kidney gives it a response-time advantage for its vital roles in management of fluid volume, electrolyte balance, pH balance, and other non-excretionary functions; where adjustments to the blood plasma volume and ionic content are quickly reflected in the interstitial fluids throughout the body to affect osmotic pressures.
*Estimate based on: Cardiac output 5 liters/minute * 20% renal flow * 50% plasma * 25% glomerular filtration rate * 60 minutes/hour * 24 hours/day = 180 liters/day
The following video provides a good overview of kidney and nephron anatomy with basic descriptions of the function of component in the overall renal system.
Video: Khan Academy – The Kidney and Nephron
Nephron Processes
The overall scheme for removing waste products from the blood is sometimes described as the “desk drawer analogy” – one approach for cleaning out a desk drawer is to dump all of drawer contents (ultrafiltration), put back all the things you want to keep (reabsorption, secretion), and discard what remains (excretion). For an overview of the nephron structure, and an introduction to where these processes take place within the nephron, review the video links below. For a more detailed look at these three sequential processes of the nephron (ultrafiltration, reabsorption, and secretion), review the videos under each process link.
Video: Armando Hasudungan, Nephrology – Kidney Physiology Overview
www.youtube.com/watch?v=KWHasxDRf54
The video also identifies the major cell types within the nephron:
-
Squamish cells line the Bowman’s capsule
-
Cuboidal cells form the tubule walls
-
Podocyte cells attached to the glomerulus and adjust filtration
-
Mesangial cells contain actin to apply contraction and altering of blood of flow
-
Granular cells wrap around afferent and efferent arterioles and secrete renin – see Renin Angiotensin Aldosterone System (RAAS)
-
Macula Densa cells in the thick ascending loop of Henle near head of Bowman’s capsule (juxtamedullary junction) are chemosensors to monitor Na+ and Cl- content going into the distal convoluted tubule
The second video by the same author appears to be an earlier version, which provides an additional overview of cell types in the last 2 minutes of the video.
Video: Armando Hasudungan, Nephrology – Kidney and Nephron Overview
www.youtube.com/watch?v=Ioir82UA9x4
Outline of video content:
-
Renal Corpuscle
-
Glomerular capsule – parietal cells, epithelial like
-
Glomerulus – podocytes
-
-
Proximal convoluted tubules
-
Cuboidal cells with microvilla
-
-
Loop of Henle
-
Thin segmented cells (epithelial-like)
-
-
Distal convoluted tubules
-
Cuboidal cells – reabsorption and secretion
-
-
Collecting duct
-
Cuboidal principle cells
-
Cuboidal intercalating cells
-
Ultrafiltration – Glomerular Filtration
The afferent arteriole provides blood to the glomerulus, which then exits through the efferent arteriole. The glomerulus is a high pressure capillary bed inside the Bowman’s capsule which separates the smaller components of plasma from the blood flow by allowing the plasma and small contents to leak through the fenestrated capillary walls (in a controlled manner) while retaining the blood cells and large proteins.
Attached to the glomerulus are podocytes which have inter-locking finger-like projections called pedicels, which form a fine meshwork around the glomerular capillaries. The gaps between the pedicels are "filtration slits" which completely block at least restrict passage of large molecular weight proteins. The larger the molecule, the less likely it is to pass through the filtration device, with an upper limit of approximately 70,000 (serum albumins) [ http://faculty.etsu.edu/currie/filtreab.htm ]. In addition to assisting with filtration, the podocytes wrap around the glomerular capillaries to add structure, allowing the network to withstand pressures about twice as high as encountered by any other capillaries in the body. The filtrate that is formed is isotonic with plasma, or approximately 300 mOsm.
There is very little pressure drop across the glomerulus. [Feher, page 640] cites Starling forces of PGC = 60 mm Hg in the glomerulus capillary, PBS = 20 mm Hg in the Bowman’s space, and πGC = 30 mm Hg osmotic pressure in the Glomerular capillary resulting in a net driving force of about 10 mm Hg in favor of filtration. The table below [http://faculty.etsu.edu/currie/filtreab.htm ] shows how the filtration force varies in the glomerulus primarily as the colloid osmotic (oncotic) pressure changes. Thus, Site 1 (blood entering the glomerulus) promotes rapid filtration (+17 mmHg); but filtration has almost ceased at Site 2 (+2 mmHg, blood exiting the glomerulus) and conditions are optimal for re-absorption at Site 3 (peritubular capillaries) around the proximal convoluted tubules (-22 mmHg).
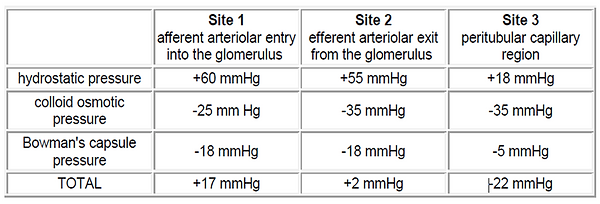
Reabsorption – Tubular Reabsorption
This section was adapted primarily from [http://faculty.etsu.edu/currie/filtreab.htm ] to provide an overview of the various reabsorption processes along the nephron. The bullet outline format is used to collect points of interest. Video links follow to provide more details.
-
Approximately 99% of filtrate is reabsorbed.
-
Urine output is about 1.5 L per day but can fall as low as 400 mL/day to conserve water, or increase dramatically to rid the body of excess water.
-
When urine output falls into the 400-600 mL/day range, it is nearly impossible to maintain a normal electrolyte and pH balance.
-
Approximately 65% of salt and water re-absorption occurs in the proximal tubule. Since the proximal tubule is freely permeable to salt and water, this reabsorption does not significantly alter the concentration of the filtrate.
-
Water follows sodium transfer in the proximal tubule, but osmolarity stays the same.
-
Both Cl- and H2O passively follow the Na+ (the H2O follows Na+ in the form of a sphere of hydration while Cl-follows Na+ because of the charge attraction).
-
Glucose and proteins/amino acids are absorbed only in the proximal tubule (there are no proteins or glucose in the loop of Henle in the normal kidney).
-
-
Approximately 20% of the renal filtrate is reabsorbed in the loop of Henle.
-
The distal convoluted tubule is permeable to water, but the permeability if regulated by ADH control of aquaporin sites.
-
Antidiuretic hormone (ADH) is synthesized in the hypothalamus, but is stored and released from the posterior pituitary. ADH also causes vasoconstriction and is part of the RAAS.
-
-
In distal tubule, K+ and creatinine are excreted, and calcium is reabsorbed.
-
The collecting duct passes through the molarity gradient in the medulla (described in the following paragraph). If ADH has caused an increase in the number of functional water channels in the collecting duct, additional water will be reabsorbed into the bloodstream and the filtrate will be further concentrated.
The renal medulla essentially functions as a “salt bed” (hypertonic) with increasing ionic concentration toward the center of the kidney which reaches a peak of approximately 1200 mOsm. Key features of this structure include:
-
The descending limb of the loop of Henle concentrates solutes in so it will be easier for the ascending limb to pump those solutes into the interstitial fluid of the medulla.
-
Permeable to water
-
-
The ascending limb concentrates solutes in the medulla.
-
Impermeable to water
-
Permeable to Na+, Cl-, and K+
-
-
The "symbiotic functional relationship" between the ascending and descending limb is known as the countercurrent multiplier system.
-
The vasa recta capillary beds mimic the prevailing concentration gradient in the kidney tubules to help trap solutes in the medullary region and maintain the high solute concentration.
-
The vasa recta are freely permeable to salt and water.
-
Solutes diffusing out of the ascending limb on the way up diffuse back into the descending limb and are carried back down.
-
Blood flow through the vasa recta is slow to allow time for equilibration of the steep concentration gradients.
-
-
In order to produce concentrated urine, the medulla must have a high solute concentration.
-
The longer the loops and the greater the number of loops; then the more time, distance and surface area available to create gradients and result in greater ability to produce concentrated urine.
Video: Khan Academy, Secondary Active Transport in Nephron
www.youtube.com/watch?v=czY5nyvZ7cU
Outline of video content:
-
Proximal tubule has “brush border” facing the lumen (apical side)
-
Peritubular capillaries surround the tubule
-
Proximal convoluted tubule reabsorbs glucose, amino acids, Na+ (ATP-driven active transport)
-
Loop of Henle reabsorbs Na+, K+, Cl- (NKC co-transporter, a secondary active transporter driven by Na+ gradient)
-
Distal tubule reabsorbs Ca2+ (Na-Ca anti-porter, a secondary transporter driven by Na+ gradient)
Video: Andrew Wolf, Renal Physiology: Reabsorption and Excretion
www.youtube.com/watch?v=uKj4PwMic7E
Outline of video content:
-
Vasa recta lined with endothelial cells, basement membrane
-
Basal side of cuboidal cells on capillaries, apical surface pointing into lumen of tubule
-
In cuboidal cells:
-
Basal surface – Na-K-ATPase pump: 3 Na+ out, 3 K+ in (requires ATP, O2, glucose)
-
Active pump sets up Na+ gradient that drives Na+ transfer at apical surface
-
-
Apical surface – Na-Glucose co-transporter: Na+ in, Glucose in
-
Apical surface – Na-K-Cl- co-transporter: Na+ in, K+ in, 2 Cl- in
-
Apical surface – Na-H counter-transport: Na+ in, H+ out (acid-base balance)
-
Apical surface – protein fragments phagocytosis
-
Video: Andrew Wolf, Concentration of Urine
www.youtube.com/watch?v=8Ssh4c9cJf0
Outline of video content:
-
Osmolarity gradient created by loop of Henle
-
Filtrate enters isotonic at 300 mOsm
-
Filtrate leaves hypotonic at 200 mOsm
-
Maximum at the bottom of the loop of Henle 1200 mOsm
Armando Hasudungan, Pharmacology - Diuretics
www.youtube.com/watch?v=NzdvoGZquIk
Outline of video content:
-
Reduce blood pressure by increasing excretion of Na and water in urine
-
Proximal tubule
-
65-70% of Na reabsorbed
-
Bicarbonate reabsorbed as part of the pH control system
-
-
Distal tubule
-
5% of Na reabsorbed, Cl
-
-
Thick descending limb
-
Water
-
-
Thick ascending limb
-
25% Na reabsorbed, K,Cl
-
-
Collecting duct
-
1-2% of Na reabsorbed
-
Water can also be reabsorbed
-
-
Osmotic diuretics
-
In Proximal and Loop of Henle
-
Increases solute concentration within the tubules, and thus causes more water to be retained
-
Decrease plasma volume, decrease blood pressure
-
-
Loop diuretics
-
Works at thick ascending limb
-
Prevent Na reabsorption (inhibits active transport of Na-K-ATPase)
-
-
Thiazide diuretics
-
Works at distal convoluted tubule
-
Inhibits Na-Cl transporter
-
-
Potassium Sparing diuretics
-
Works at distal end of distal tubule and collecting duct
-
The Glomerular Filtration Rate ( GFR = Kf [PGC - PBS - πGC] ) is regulated by the 4 parameters shown, as noted in [Feher, pages 665-666).
-
PBS : The pressure in Bowman’s space is approximately 20 mm Hg and does not vary much because it is a function of structure and compliance.
-
πGC : If water is lost due to dehydration, the blood becomes more concentrated and oncotic pressure increases. This decreases GFR, and reduces further loss of fluid through the urine. This can only be restored by drinking water.
-
PGC : GFR can be adjusted up or down by constriction/dilation of either the afferent or efferent arteriole.
-
Kf : The filtration coefficient is regulated at the glomerulus.
Cardiac Output (CO) is approximately 5000 ml/minute, and about 20% of that goes to the kidneys = 1000 ml/minute.
-
Renal Plasma Flow (RPF) is approximately half, or 500 ml/minute, depending on the percentage of plasma in the blood.
-
Glomerular Filtration Rate (GFR ) = Renal Plasma Flow * Filtration Fraction is approximately 125 ml/minute.
Plasma Flow can be increased by increasing cardiac output, or by increasing renal flow by vasodilation of the afferent arterioles. The Filtration Fraction can be increased by vasoconstriction of the efferent arterioles.
Video: Armando Hasudungan, Nephrology – Glomerular Filtration
www.youtube.com/watch?v=8Mn0IUCTg3U
Outline of video content:
-
Filtration Fraction – approximately 20% of plasma volume is filtered by the glomerulus
-
Podocytes and basement membrane have overall negative charge => big negatively charged proteins do not pass through
-
Filtration pressure causes movement outward
-
PGH Glomerular hydrostatic pressure +60 mm Hg (blood pressure)
-
Bowman’s capsule pressure -16 mm Hg
-
Glomerular colloid osmotic pressure -34 mm Hg
-
Net ~ +10 mm Hg in filtration direction
-
-
Glomerular Filtration Rate (GFR) = Kf * Net Filtration Rate is 125 ml/min or 180 L/day
-
Kf = coefficient based on surface filtration area of capillary and permeability
-
Net Filtration Pressure = 10 mm Hg – this can be altered
-
GFR is an indicator of kidney function
-
PGH decreased by restriction of afferent arteriole
-
PGH increased by restricting efferent arteriole, or dilation of afferent arteriole
Secretion – Tubular Secretion
Secretion is movement of substances from the blood in the peritubular capillaries into the tubules for excretion. This topic was included in the reabsorption processes, but this section collects other more specific secretion topics.
Substances targeted for secretion/excretion include:
-
H+, uric acid, and ammonium (see ph Balance)
-
Organic ions such as bile salts, food additives (such as benzoate, saccharine)
-
Salicylate (aspirin), and drugs such as penicillin and antihistamine are secreted using facilitated transport, the organic ion transporter (OAT).
Video: Dr. Dan McCann, Lect 26: Renal Tubular Reabsorption and Secretion (last part)
www.youtube.com/watch?v=t9wO9Rx270c
The renal threshold for phosphate (HPO4-2 and H2PO4-1) is about 1 mM [Feher, page 648]. Increasing plasma phosphate concentration above the threshold increases the amount of phosphate that is excreted. The rate is linear, proportional to the GFR.
-
Parathyroid hormone (PTH) is secreted by the parathyroid glands in response to hypocalcemia (low plasmas calcium concentration). The increased PTH increases bone resorption that increases both calcium and phosphate in the plasma. PTH also decreases the renal threshold for phosphate so that more phosphate is excreted in order to raise the calcium concentration without increasing the plasma phosphate concentration.
Kidneys normally do not participate in the regulation of plasma glucose concentration [Feher, page 647].
Diagnostic agents
-
Inulin is a polysaccharide produced by many types of plants. Inulin is not secreted or reabsorbed in any appreciable amount at the nephron. The concentration of inulin in the ultrafiltrate is the same as its concentration in the plasma. Thus, inulin (and its analog sinistrin) are used to help measure kidney function by calculation of the glomerular filtration rate (GFR). However, due to clinical limitations, inulin and sinistrin are rarely used for this purpose and creatininevalues are the standard for determining an approximate GFR [Feher, pages 635 and 649; and http://en.wikipedia.org/wiki/Inulin ]
-
Para-aminohippuric acid (PAH) is used in the measurement of renal plasma flow. It is not naturally found in humans, and must be IV infused before use diagnostically. PAH differs from inulin in that the fraction of PAH that bypasses the glomerulus and enters the nephron's tubular cells (via the peritubular capillaries) is completely secreted. Thus, renal venous blood contains very little PAH. Renal clearance of PAH is useful in calculation of renal plasma flow (RPF) = (1 − hematocrit) × renal blood flow. The clearance of PAH, however, is reflective only of RPF to portions of the kidney that deal with urine formation, and thus underestimates actual RPF by about 10%. [Feher, page 635; and http://en.wikipedia.org/wiki/Aminohippuric_acid ]
Water Balance
Stretch receptors in the walls of the left atria and intrathoracic veins release ADH in response to decreased cardiac preload (low venous volume). Reduction of ADH in the kidney activates aquaporins AQP2 in the nephron late distal tubule and in the collecting duct to recover water from the urine filtrate. ADH also increases urea permeability in the inner medullary collecting duct, which results in a higher osmotic gradient. Thus, water is conserved to maintain the blood plasma volume. [Feher, page 670]
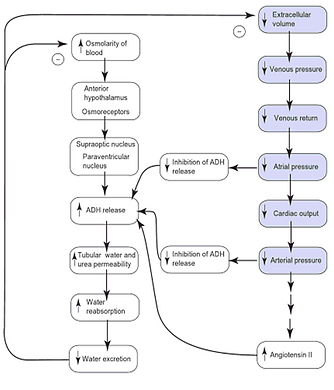
For a broader view the ADH secretion system, see
Video: Khan Academy – ADH Secretion
www.youtube.com/watch?v=8dQdfbE8kFU&list=PLgLExqo2buqvlQIPcD44ImjaxHLR-9ntU&index=7
Video: Khan Academy – ADH Effects on Blood Pressure
www.youtube.com/watch?v=Ot1We4_EfRE&index=8&list=PLgLExqo2buqvlQIPcD44ImjaxHLR-9ntU
Renin-Angiotensin-Aldosterone System
The Renin-Angiotensin-Aldosterone System (RAAS) regulates Na+ balance and blood pressure. Renin is released by granule cells in the afferent arteriole. Renin converts angiotensinogen into angiotensin I. Angiotensin converting enzyme (ACE) performs the conversion to angiotensin II. Angiotensin II causes multiple actions to increase the extracellular fluid volume:
-
Vasoconstriction, which increases blood pressure
-
Release of aldosterone from the adrenal gland at the top of the kidney, which reduces loss of Na+
-
Thirst sensation, to signal the need for fluid intake
-
ADH release, to recapture filtered water
-
Increased reabsorption of Na+ and HCO3- in the proximal convoluted tubule
The juxtaglomerular apparatus refers to the arrangement of descending loop adjacent to both the afferent and efferent arterioles at the point where they enter and exit the glomerulus. This location is where the thick ascending limb ends and the distal convoluted tubule begins. The juxtaglomerular apparatus regulates afferent arteriole pressure in response to sensing [NaCl] by the macula densa. The macula densa can constrict the afferent arteriole and release renin.
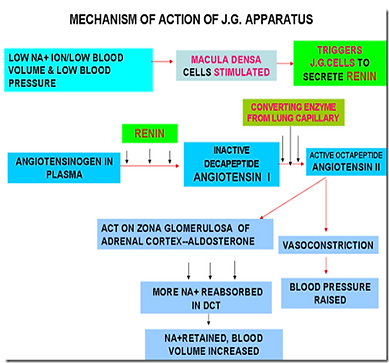
Video: Khan Academy series – the title of each video will help you find the specific topic of interest.
Khan Academy – General Overview of the RAAS System
www.youtube.com/watch?v=BVUeCLt68Ik
Khan Academy – Renin Production in Kidneys
www.youtube.com/watch?v=AtlhAhONHyM
Khan Academy – ADH Secretion
www.youtube.com/watch?v=8dQdfbE8kFU
Khan Academy – Angiotensin 2 Raises Blood Pressure
www.youtube.com/watch?v=Oges5YqDLHY
Khan Academy – Activating Angiotensin 2
www.youtube.com/watch?v=cUVS0AlRyw8
Khan Academy – Aldosterone and ADH
www.youtube.com/watch?v=Z0XPMFL45oo
Khan Academy – Aldosterone Raises Blood Pressure and Lowers Potassium
www.youtube.com/watch?v=64_ZfIemRGM
Video: Armando Hasudungan, Renin-Angiotensin System (Renin, Prorenin)
www.youtube.com/watch?v=ZVQd21fb1Mk
Andrew Wolf, Renin Angiotensin Aldosterone System (RAAS) Physiology
www.youtube.com/watch?v=IrPLzVX04q4
Outline of video content:
-
Brain senses drop in blood pressure
-
Sympathetic β1 adrenic receptor on kidney by epinephron or noreprinephron (from nervous system adrenals)
-
Juxtaglomerular cells around afferent arteriole produce rennin
-
Distal tubule macula densa cells sense low blood pressure (low sodium or low chloride), signal juxtaglomerular cells to secrete rennin
-
Juxtaglomerular cells around afferent arteriole produce pro-renin, which is renin with additional amino acids that block the activation site (cleft)
-
Juxtaglomerular cells are sensitive to stretch, and sense arteriole pressure
-
Synthesize and secrete renin
-
Decreased kidney flow decreases stretch, which decrease glomerular flow (changes Na, Cl in distal tubule)
-
-
Podocytes and mesengial cells help convert pro-renin to renin
-
PRR receptors (pro-renin receptors)
-
Binding and cleaving of the additional amino acids converts to renin
-
-
Angiotencin II
-
Stimulates adrenal cortex, secretes aldosterone, causes kidneys to reabsorb and retain Na and Cl (cause rise in blood pressure)
-
Stimulates pituitary gland to secrete ADH (anti-diuretic hormone), causes water retention in kidney,
-
Stimulate hypothalamus to stimulate thirst center, consume more water
-
Stimulates heart, causing cardiac hypertrophy (long term)
-
Causes arterial constriction, increases blood pressure
-
-
Renin activates angiotensinogen (from liver), converts it to angiotensin I (A1)
-
A1 converted to A2 by ACE (angiotensin conversion enzyme) released in lungs (primarily)
-
ACE also inhibits bradykinin (vaso dialator)
-
A2 causes kidney to produce aldosteone
-
Increases reabsorption of Na, increases fluid, increases blood pressure
-
Decreases K
-
-
A2 causes vasoconstriction of kidney arterioles
Ph Balance
Regulation of pH by secreting more or less H+ is managed based on the HCO3- filtered by the glomerulus and transferred to the blood. CO2 from the plasma is hydrated to form H2CO3 (catalyzed by carbonic anhydrase), which dissociates to HCO3- and H+. The HCO3- is moved to the blood stream by the Na+- HCO3- cotransporter. In the α-intercolated cell, the H+ is moved across the apical membrane to the tubule by one of three mechanisms: H+-ATPase active transporter, a Na+-H+ cotransporter, or a H+-K+ATPase transporter. The activity of the first two transporters is increased by aldosterone.
Video: Kahan Academy – Aldesterone removes acid from the blood
www.youtube.com/watch?v=7HTy-6NLWGM&list=PLgLExqo2buqvlQIPcD44ImjaxHLR-9ntU&index=6
Outline of video content:
-
α-intercalated cells secrete acid
-
β-intercalated cells secrete base
-
Aldosterone activates ATP-driven transporter
-
NaCl gradient transporter also enhanced by aldosterone
-
Na+ H+ cotransfer in tubule
-
HCO3- is filtered by the glomerulus
-
HCO3- reacts with H to produce CO2
-
CO2 diffuses out and is reacted with carbonic anhydrase, breaks down to HCO3- and H+
-
HCO3- goes back into the bloodstream
-
Cycle repeats
-
Other non-excretionary functions
Erythropoietin
Erythropoietin is produced in the kidney and secreted into the blood in response to hypoxia [Feher, page 432]. The reticuloendothelial system includes macrophages that remove damaged erythrocytes, which must be continually replaced in order to maintain the O2 delivery capacity. The oxygen level of the blood, as sensed by the kidney, drives the release of erythropoietin to stimulate stem cells in bone marrow to produce new erythrocytes.
Gluconeogenesis
Glucose can be produced by the kidney in times of starvation by converting amino acids to glucose.
Activation of Vitamin D
Vitamin D is produced in the skin by exposure to light, but must be activated by two hydroxylation reactions. The first occurs in the liver to produce 25-O-cholecalciferol. The second reaction occurs in nephron proximal tubule cells to produce the circulating form of Vitamin D,1,25-(OH)2 cholecalciferol. The reaction in the kidney is simulated by parathyroid hormone (PTH) and low plasma phosphorous concentration. This helps maintain plasma concentrations of both calcium and phosphorous. [Feher, page 631]
P.S. Reminder that this real, not just anatomy and physiology cartoons...

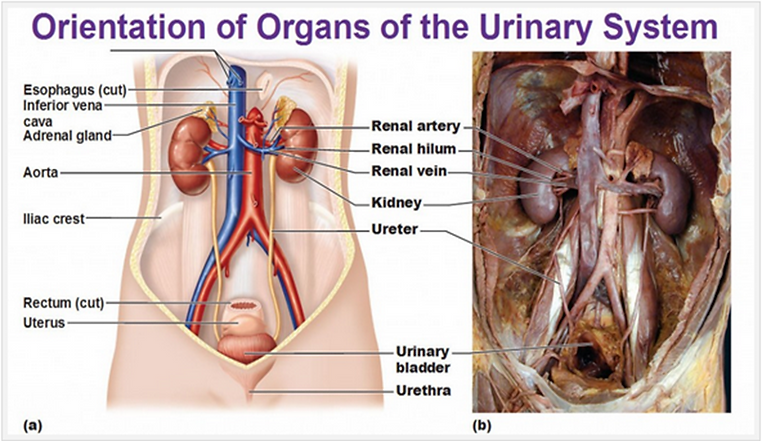
Microscopy
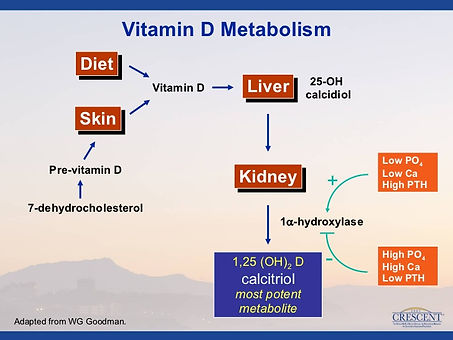
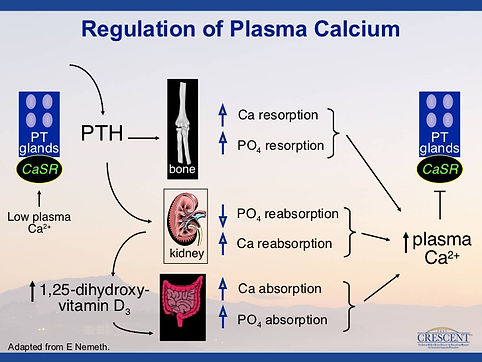
Degradation of Hormones
The kidney participates in the degradation of angiotensin II, parathyroid hormone (PTH), insulin, and glucagon. The degradation is proportional to hormone concentration. This system independently counters the hormone secretion systems to maintain balance. [Feher, page 631]
Calcium Regulation
If the kidney is not able to regulate calcium levels, then calcium is removed from bone to maintain blood levels. This can lead to skeletal changes.

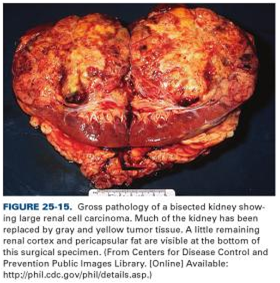

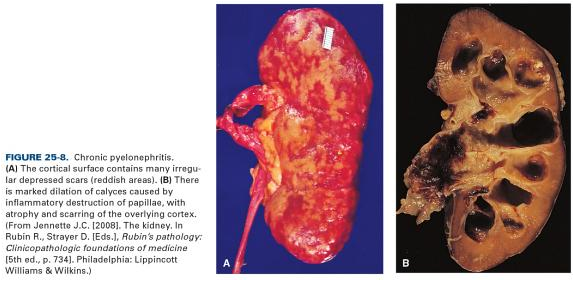
Porth, page 629
Porth, page 636
Porth, page 638
Porth, page 638